Enhance neurological recovery with cutting-edge AI and rehabilitation robotics. Discover how advanced technologies synergize to create personalized, effective, and efficient rehabilitation programs, fostering improved motor skills and cognitive functions. Explore the intersection of AI algorithms and robotic assistance, revolutionizing the landscape of neurological rehabilitation for a brighter path to recovery.
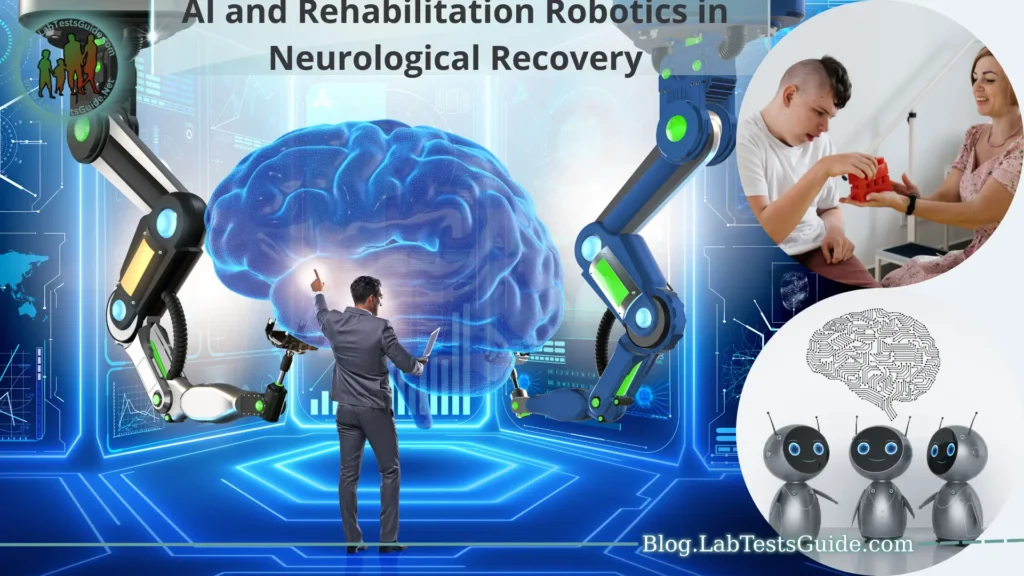
This document delves into the intersection of AI and rehabilitation robotics, exploring how these cutting-edge technologies collaborate to restore function and enhance the lives of individuals affected by neurological injuries. We will navigate through the landscape of neurological injuries, their consequences, and the limitations of conventional rehabilitation techniques. By delving into the evolution of rehabilitation robotics and the burgeoning role of AI in healthcare, we will uncover the potential for synergistic advancements that have the power to reshape the rehabilitation paradigm. Through insightful case studies, innovative approaches, and forward-looking discussions, we aim to provide a comprehensive understanding of the impact of AI and robotics on neurorehabilitation, shedding light on the path towards improved recovery and a brighter future for those facing neurological challenges.
What is the Role of Artificial Intelligence in Revolutionizing Neurological Rehabilitation?
- Personalized Treatment Plans: AI analyzes patient data, such as neuroimaging and movement patterns, to tailor rehabilitation plans that suit individual needs and adapt over time.
- Early Detection and Assessment: AI algorithms can identify subtle changes in neurological conditions, enabling early detection and timely intervention for better outcomes.
- Predictive Modeling: By analyzing large datasets, AI can predict recovery trajectories, helping clinicians set realistic goals and optimize treatment strategies.
- Enhanced Brain-Computer Interfaces (BCIs): AI improves the accuracy of BCIs, enabling direct communication between the brain and external devices for patients with limited mobility.
- Real-time Feedback: AI-powered sensors provide instant feedback during rehabilitation sessions, ensuring correct movement patterns and motivating patients through gamification.
- Adaptive Robotic Assistance: Robots with AI algorithms can adapt assistance levels based on the patient’s progress, challenging them appropriately for optimal recovery.
- Cognitive Rehabilitation with Virtual Reality: AI-driven virtual reality applications offer engaging cognitive exercises that adjust difficulty levels based on the patient’s performance.
- Data-Driven Insights: AI analyzes patient data to uncover patterns, allowing clinicians to make informed decisions and refine treatment plans for better outcomes.
- Remote Monitoring and Telehealth: AI enables remote monitoring of patients’ progress, allowing clinicians to adjust therapies in real-time and extending rehabilitation beyond clinical settings.
- Reduced Healthcare Costs: AI-driven rehabilitation can lead to shorter hospital stays, fewer readmissions, and overall cost savings by optimizing treatment efficiency.
- Continuous Learning and Improvement: AI algorithms learn from each patient interaction, contributing to a growing knowledge base that benefits future rehabilitation efforts.
- Research Acceleration: AI aids researchers in analyzing vast amounts of data, accelerating the development of new therapies and understanding the intricacies of neurological recovery.
- Customized Assistive Devices: AI designs and tailors assistive devices, such as exoskeletons, to match the patient’s biomechanics and provide targeted support.
- Ethical Considerations: AI introduces discussions on patient consent, privacy, and data security, prompting ethical frameworks to balance technological advancements with patient rights.
How to Implement AI-Powered Robotic Therapies for Optimal Neurological Rehabilitation Outcomes:
Needs Assessment and Goal Setting:
- Identify the specific neurological conditions you aim to address through AI-powered robotic therapies.
- Set clear rehabilitation goals, such as improving motor function, enhancing cognitive abilities, or facilitating communication.
- Determine the target patient population and assess their individual needs and preferences.
Data Collection and Integration:
- Gather comprehensive patient data, including medical history, neuroimaging, and movement patterns.
- Integrate data from wearable sensors, IoT devices, and other sources to provide real-time insights into patient progress.
AI Algorithm Development:
- Collaborate with experts in AI and machine learning to develop algorithms tailored to your rehabilitation goals.
- Train AI models using diverse datasets to enable accurate assessment, prediction, and customization of therapies.
Robotic Device Selection and Integration:
- Choose suitable robotic devices, such as exoskeletons, prosthetics, or brain-computer interfaces, based on the targeted neurological conditions.
- Ensure seamless integration of AI algorithms with the chosen robotic devices for effective real-time interaction.
Customization of Rehabilitation Protocols:
- Develop personalized treatment protocols that leverage AI insights to adapt therapies according to individual patient progress.
- Implement AI-driven algorithms to adjust assistance levels, task difficulty, and session duration based on real-time feedback.
User Interface and Experience Design:
- Design intuitive user interfaces that facilitate patient interaction with AI-powered robotic systems.
- Focus on user experience to ensure patients find the therapies engaging, motivating, and easy to use.
Clinical Validation and Testing:
- Conduct rigorous clinical trials to assess the safety, efficacy, and feasibility of AI-powered robotic therapies.
- Collaborate with medical professionals to gather qualitative and quantitative data on patient outcomes.
Continuous Monitoring and Feedback Loop:
- Implement systems for continuous monitoring of patient progress both in clinical settings and remotely.
- Utilize AI analytics to provide regular feedback to clinicians, allowing them to refine and optimize treatment plans.
Clinician Training and Education:
- Train healthcare professionals in using AI-powered robotic therapies effectively.
- Provide resources and support for clinicians to interpret AI-generated insights and make informed decisions.
Ethical Considerations and Patient Consent:
- Establish clear protocols for obtaining patient consent for AI-driven therapies and data usage.
- Address ethical concerns related to privacy, data security, and patient autonomy.
Long-term Engagement and Maintenance:
- Foster patient engagement through ongoing communication, education, and support.
- Regularly update AI algorithms and robotic systems based on new research findings and patient feedback.
Collaboration and Knowledge Sharing:
- Collaborate with interdisciplinary teams, including neurologists, engineers, therapists, and ethicists, to ensure a holistic approach.
- Contribute to the broader healthcare community by sharing insights, research findings, and best practices.
Neurological Injuries: Causes and Consequences:
Neurological injuries, stemming from a variety of causes, can significantly impact an individual’s nervous system, leading to a range of debilitating consequences. These injuries can disrupt fundamental functions of the brain and spinal cord, resulting in profound physical, cognitive, and emotional challenges. This section explores the causes behind neurological injuries and delves into the far-reaching consequences that individuals facing these injuries often encounter.
Causes of Neurological Injuries:
- Trauma and Accidents: Motor vehicle accidents, falls, sports injuries, and physical trauma can result in damage to the brain or spinal cord, causing neurological impairments.
- Vascular Incidents: Conditions like strokes, caused by disrupted blood flow to the brain, can lead to neurological injuries. Ischemic strokes result from blood vessel blockages, while hemorrhagic strokes involve bleeding in the brain.
- Degenerative Disorders: Neurodegenerative diseases, such as Alzheimer’s, Parkinson’s, and multiple sclerosis, involve progressive nerve cell deterioration, leading to neurological deficits.
- Infections and Inflammation: Infections like encephalitis, meningitis, and autoimmune disorders can trigger inflammation that damages nervous tissue.
- Tumors and Growth Abnormalities: Brain tumors, both benign and malignant, as well as abnormal growths like arteriovenous malformations (AVMs), can compress or disrupt neural structures.
- Genetic Factors: Inherited genetic mutations can contribute to conditions like Huntington’s disease, muscular dystrophy, and various hereditary neuropathies.
Consequences of Neurological Injuries:
- Motor Dysfunction: Neurological injuries often result in impaired motor control, causing difficulties in movement, coordination, and muscle strength.
- Sensory Impairments: Individuals may experience altered sensations, reduced sensitivity, or complete loss of sensory perceptions, affecting touch, pain, temperature, and proprioception.
- Cognitive Challenges: Neurological injuries can lead to memory problems, difficulties in concentration, impaired reasoning, and altered cognitive processing.
- Communication Limitations: Language and communication skills may be affected, leading to challenges in expressing thoughts, understanding speech, and social interaction.
- Emotional and Behavioral Changes: Individuals might undergo mood swings, depression, anxiety, impulsivity, and personality alterations due to neurological damage.
- Functional Independence: Daily activities such as self-care, mobility, and managing daily tasks can become challenging, impacting overall independence.
- Quality of Life Impact: Neurological injuries often lead to reduced quality of life due to limitations in physical abilities, cognitive functions, and social engagement.
- Pain and Discomfort: Chronic pain, neuropathic pain, and other sensory disturbances are common consequences of neurological injuries.
Rehabilitation Robotics: An Overview:
Rehabilitation robotics represents a pioneering field that fuses cutting-edge technology with healthcare to enhance the recovery and rehabilitation process for individuals facing physical and neurological impairments. By integrating robotic systems into traditional rehabilitation approaches, this multidisciplinary field aims to optimize patient outcomes, restore lost function, and improve overall quality of life. This section provides an insightful overview of rehabilitation robotics, exploring its evolution, various types of robotic devices, and the potential benefits and limitations it brings to the realm of rehabilitation.
Evolution of Rehabilitation Robotics:
The inception of rehabilitation robotics can be traced back to the mid-20th century, with the development of devices like the “Gait Trainer” and the “MIT-Manus” robotic arm. These early endeavors set the stage for the integration of robotic technologies into rehabilitation therapies, paving the way for innovative advancements over the years.
Types of Rehabilitation Robots:
- Exoskeletons: These wearable robotic devices are designed to assist or enhance limb movement, providing support, resistance, or guidance during exercises and activities.
- End-Effector Robots: Devices that focus on interacting with the limb’s endpoint (such as the hand or foot), enabling a range of movements and exercises.
- Robotic Prosthetics: Artificial limbs equipped with sensors and actuators to mimic natural movements, aiding amputees in regaining functional mobility.
- Assistive Robots: Robotic systems that provide assistance with daily activities, such as lifting, transferring, and mobility support.
- Interactive Gaming Systems: Utilizing virtual reality and gamification, these systems engage patients in enjoyable activities that also promote physical and cognitive recovery.
Benefits and Limitations of Rehabilitation Robotics:
Benefits:
- Personalized and Intensive Therapy: Rehabilitation robots offer tailored and intensive therapy sessions that can be adjusted to an individual’s progress and needs.
- Consistent Assistance: Robots provide consistent, reliable assistance, reducing the strain on therapists and ensuring accurate movement patterns.
- Data-Driven Insights: Robotic systems generate vast amounts of data, enabling clinicians to track progress, analyze performance, and make informed decisions.
- Motivation and Engagement: Gamified and interactive approaches enhance patient motivation and engagement, leading to better adherence to therapy protocols.
Limitations:
- High Initial Costs: The development and implementation of rehabilitation robots can be financially demanding, limiting their availability.
- Lack of Human Touch: Some patients value the human connection in rehabilitation, which robotic systems may struggle to replicate.
- Complexity and Training: Operating and maintaining robotic devices require specialized training for both clinicians and patients.
- Limited Customization: While increasingly flexible, rehabilitation robots might not fully adapt to each patient’s unique needs.
Integration of AI in Neurorehabilitation:
The integration of artificial intelligence (AI) into neurorehabilitation represents a groundbreaking convergence of technology and healthcare, revolutionizing the way individuals recover from neurological injuries. By harnessing AI’s analytical prowess and predictive capabilities, neurorehabilitation becomes a more personalized, data-driven, and effective process. This section delves into the multifaceted role of AI in neurorehabilitation, exploring its applications, advantages, and the transformative potential it offers to enhance recovery outcomes.
Role of Artificial Intelligence in Healthcare:
Data Analysis and Pattern Recognition: AI processes vast amounts of patient data, such as neuroimaging scans and movement profiles, to detect subtle patterns and changes that might go unnoticed by traditional methods.
- Predictive Modeling: AI algorithms can forecast recovery trajectories based on individual patient characteristics, enabling clinicians to set realistic goals and tailor treatment plans.
- Personalized Treatment Planning: AI-driven analysis allows for the customization of rehabilitation strategies, optimizing interventions to address each patient’s unique needs.
- Real-time Monitoring: AI-powered sensors provide real-time feedback during rehabilitation sessions, aiding both patients and therapists in adjusting exercises for optimal progress.
- Adaptive Interventions: AI algorithms adapt rehabilitation protocols on-the-fly, modifying task difficulty and assistance levels based on real-time performance data.
AI Applications in Neurorehabilitation:
- Brain-Computer Interfaces (BCIs): AI enhances the precision of BCIs, enabling direct communication between the brain and external devices, facilitating movement in patients with severe impairments.
- Neuroimaging and Injury Assessment: AI aids in the analysis of neuroimaging data, assisting clinicians in diagnosing injuries, assessing damage, and tracking recovery progress.
- Virtual Reality and Gamification: AI-driven virtual reality applications offer engaging environments for cognitive and motor exercises, tailoring difficulty levels to challenge and motivate patients.
- Data-Driven Insights: AI analytics uncover correlations and trends within patient data, guiding clinicians in refining treatment plans and making informed decisions.
Machine Learning and Data Analysis in Rehabilitation:
- Gait Analysis and Movement Rehabilitation: AI algorithms analyze movement patterns, facilitating targeted interventions to improve gait and motor skills.
- Cognitive Training and Rehabilitation: AI-powered cognitive exercises adapt to individual performance, enhancing memory, attention, and problem-solving abilities.
- Predicting Recovery Trajectories: Machine learning models assess patient data to predict recovery trajectories, helping therapists anticipate challenges and adjust strategies.
- Remote Monitoring and Telehealth: AI enables remote patient monitoring, allowing therapists to track progress, provide guidance, and adjust therapies from a distance.
AI-Driven Assessment and Diagnosis:
The application of artificial intelligence (AI) to assessment and diagnosis in neurorehabilitation has ushered in a new era of precision and insight, revolutionizing the way neurological injuries are understood and treated. By leveraging AI’s computational capabilities and data analysis, healthcare professionals can achieve more accurate and timely assessments, leading to improved prognoses and tailored treatment strategies. This section delves into the pivotal role of AI-driven assessment and diagnosis in neurorehabilitation, highlighting its key applications, benefits, and the transformative impact it brings to patient care.
Neuroimaging and Injury Assessment:
- Image Analysis and Segmentation: AI algorithms analyze neuroimaging data, segmenting brain structures and lesions with high precision, aiding in the diagnosis and quantification of injuries.
- Early Detection: AI identifies subtle changes in neuroimaging scans, allowing for early detection of neurological injuries and providing opportunities for timely intervention.
- Predicting Recovery Trajectories: AI analyzes neuroimaging data to predict recovery trajectories, assisting clinicians in setting realistic expectations and tailoring treatment plans.
Personalized Treatment Planning through AI:
- Biomarker Identification: AI identifies biomarkers from patient data, aiding in the selection of appropriate interventions and tracking treatment responses.
- Treatment Customization: AI-driven analysis of patient characteristics and responses guides the customization of rehabilitation strategies, optimizing outcomes for individual needs.
- Progress Monitoring: AI continuously assesses patient progress, enabling therapists to make informed adjustments to treatment plans in real-time.
Machine Learning for Clinical Assessment:
- Cognitive Assessment: AI-driven cognitive tests adapt to individual performance, providing detailed insights into cognitive impairments and recovery progress.
- Motor Function Analysis: Machine learning algorithms analyze movement patterns to quantify motor impairments, allowing for targeted therapy interventions.
- Predictive Modeling: Machine learning models process patient data to predict recovery trajectories, assisting clinicians in anticipating challenges and adapting strategies.
Integration with Brain-Computer Interfaces (BCIs):
- Enhanced Communication: AI augments BCIs, enabling more accurate translation of neural signals into meaningful actions, facilitating communication for patients with severe disabilities.
- Motor Rehabilitation: AI-powered BCIs facilitate motor rehabilitation by interpreting neural signals to control external robotic devices or assistive technologies.
Real-time Feedback and Progress Tracking:
- Immediate Feedback: AI-powered sensors provide real-time feedback during therapy sessions, assisting patients in adjusting movement patterns and optimizing performance.
- Longitudinal Data Analysis: AI analyzes longitudinal data to track progress over time, helping therapists monitor changes and make data-driven decisions.
Robot-Assisted Neurorehabilitation Techniques:
Robot-assisted neurorehabilitation techniques represent a pioneering approach that combines advanced robotic technologies with rehabilitation strategies to enhance the recovery process for individuals grappling with neurological injuries. By providing targeted assistance, precise movement guidance, and interactive exercises, these techniques offer new avenues for restoring motor and cognitive functions. This section delves into the realm of robot-assisted neurorehabilitation, exploring various techniques, their applications, and the transformative impact they bring to the field of neurorehabilitation.
Robotic Exoskeletons for Motor Rehabilitation:
- Gait Training: Robotic exoskeletons facilitate guided walking exercises, assisting individuals with impaired lower limb function to regain walking abilities.
- Assistance and Resistance: Exoskeletons provide adjustable assistance and resistance, tailoring the level of support to match the patient’s needs and progression.
- Active Rehabilitation: Robotic devices encourage active participation by providing real-time feedback and adapting assistance levels based on the patient’s effort.
Brain-Computer Interfaces (BCIs) in Rehabilitation:
- Neural Control of Devices: BCIs interpret neural signals to control external robotic devices, enabling patients with limited mobility to interact and perform tasks.
- Motor Rehabilitation: BCIs facilitate motor recovery by translating brain signals into specific movements, encouraging neural plasticity and functional restoration.
- Communication Enhancement: BCIs enable patients with communication impairments to express themselves through direct brain-to-computer interfaces.
Virtual Reality and Gamification for Cognitive Rehabilitation:
- Cognitive Exercises: Virtual reality environments offer engaging platforms for cognitive training, enhancing memory, attention, and problem-solving skills.
- Interactive Challenges: Gamified scenarios encourage patients to perform cognitive tasks, making rehabilitation enjoyable and motivating.
- Functional Simulation: Virtual reality allows patients to practice real-life scenarios in a controlled environment, promoting cognitive and decision-making skills.
Sensor-Assisted Motor Rehabilitation:
- Real-time Feedback: Wearable sensors provide immediate feedback on movement quality and posture, aiding patients in achieving correct movement patterns.
- Data-Driven Progress Tracking: Sensor data analysis allows therapists to monitor patient progress, adjust therapies, and tailor interventions based on quantifiable metrics.
- Home-Based Rehabilitation: Sensors enable remote monitoring and home-based exercises, extending rehabilitation beyond clinical settings.
Combination Therapies and Hybrid Approaches:
- Exoskeleton-BCI Integration: Combining exoskeletons with BCIs enables coordinated limb movements through neural control, promoting holistic recovery.
- Robot-Virtual Reality Hybrid: Integrating robotics with virtual reality creates immersive rehabilitation environments that target both motor and cognitive domains.
- Customized Therapy Plans: Hybrid approaches allow therapists to design personalized interventions that address multiple aspects of neurological recovery.
Sensor Technologies and Data Integration:
Here are key points highlighting the significance of sensor technologies and data integration in neurorehabilitation.
Wearable Sensors for Real-Time Monitoring:
- Wearable sensors, such as accelerometers and gyroscopes, capture movement patterns and biomechanical data during rehabilitation sessions.
- Real-time monitoring enables immediate feedback to patients and therapists, promoting correct movement execution and enhancing engagement.
- Sensors track joint angles, muscle activation, and balance, helping quantify progress and fine-tune treatment protocols.
Objective Assessment and Progress Tracking:
- Sensor data provides objective measurements of patients’ performance, minimizing subjectivity and allowing accurate assessment of improvements.
- Quantifiable metrics help therapists track changes over time, set achievable goals, and make informed decisions for treatment adjustments.
Data Fusion and Analysis for Progress Tracking:
- Combining sensor data with other patient information, such as neuroimaging and clinical history, enables comprehensive progress tracking.
- AI-driven data fusion provides a holistic view of rehabilitation outcomes, facilitating evidence-based decisions.
Customized Treatment Plans:
- Sensor data informs therapists about patients’ movement capabilities and limitations, allowing for personalized treatment plans.
- Individualized interventions target specific deficits and adapt as patients progress, optimizing recovery outcomes.
Biofeedback and Motivation:
- Real-time biofeedback from sensors reinforces correct movement patterns, helping patients adjust and improve their motor skills.
- Visualizing progress motivates patients to actively participate and challenges them to reach higher levels of performance.
Remote Monitoring and Telehealth:
- Wearable sensors enable remote monitoring of patients’ progress outside clinical settings, extending rehabilitation to home environments.
- Telehealth platforms utilize sensor data for virtual consultations, enabling therapists to guide and adjust therapies remotely.
Big Data and Research Insights:
- Aggregated sensor data from diverse patients contribute to a rich dataset for research and analysis, leading to insights into rehabilitation outcomes.
- Large-scale data analysis uncovers trends, best practices, and novel interventions, driving continuous improvement.
- Real-world Functional Assessment: Wearable sensors allow assessment of patients’ functional abilities in real-world scenarios, aiding in designing strategies for daily life challenges.
- Immediate Adaptation of Therapies: Real-time sensor data triggers immediate adjustments to therapy parameters, ensuring that exercises remain challenging yet achievable.
- Ethical and Privacy Considerations in Data Usage: Integrating sensors requires addressing ethical concerns, ensuring patient consent, data security, and privacy throughout the data lifecycle.
Clinical Case Studies and Success Stories:
Clinical case studies and success stories serve as compelling testimonies to the transformative impact of neurorehabilitation interventions, showcasing the potential of innovative approaches, technologies, and personalized care. These real-world examples highlight the progress and achievements of individuals who have overcome neurological injuries through dedicated rehabilitation efforts. This section explores notable clinical case studies and success stories, underscoring the profound influence of neurorehabilitation on the lives of those affected.
Stroke Rehabilitation through AI-Driven Robotics:
- Case Study: A stroke survivor with severe upper limb impairment undergoes rehabilitation using a robotic exoskeleton integrated with AI algorithms. The exoskeleton assists in repetitive, targeted movement exercises while AI adjusts assistance levels based on the patient’s effort and progress.
- Outcome: Over several weeks, the patient experiences significant improvement in arm mobility and strength. The AI-driven system adapts to the patient’s evolving abilities, challenging them appropriately and enabling them to perform everyday tasks independently.
Spinal Cord Injury Rehabilitation with Brain-Computer Interfaces (BCIs):
- Case Study: A patient with a complete spinal cord injury participates in a BCI-assisted rehabilitation program. The BCI translates neural signals into control commands for a robotic arm, allowing the patient to grasp and manipulate objects in a virtual environment.
- Outcome: Through consistent training, the patient achieves remarkable dexterity with the robotic arm, translating to improved functional capabilities in real-world scenarios. The BCI-facilitated therapy not only enhances motor skills but also boosts the patient’s self-esteem and quality of life.
Pediatric Neurorehabilitation using Virtual Reality and Gamification:
- Case Study: A child with a neurological injury engages in cognitive rehabilitation using virtual reality games tailored to their cognitive abilities. The games challenge memory, attention, and problem-solving skills through interactive scenarios.
- Outcome: The child’s engagement with the virtual reality games leads to improved cognitive function, as evidenced by standardized cognitive assessments. The gamified approach makes rehabilitation enjoyable and helps the child build cognitive skills crucial for academic and social success.
Long-term Recovery with Sensor-Assisted Home-Based Rehabilitation:
- Case Study: An individual with a traumatic brain injury transitions to home-based rehabilitation using wearable sensors. The sensors track movement patterns and provide real-time feedback, enabling the patient to continue exercises independently.
- Outcome: Over the course of several months, the patient achieves gradual improvements in mobility and balance. The remote monitoring of sensor data by therapists allows for timely adjustments to therapy protocols, ensuring sustained progress.
Future Directions and Challenges:
Here are the future directions and challenges that will shape the landscape of neurorehabilitation.
Future Directions:
- Neuroplasticity and Precision Rehabilitation: Harnessing the potential of neuroplasticity through targeted interventions that promote specific neural connections for optimal recovery.
- Brain-Computer Interfaces (BCIs) Advancements: Advancing BCIs to enable more intuitive and seamless communication between the brain and external devices, unlocking new possibilities for motor and cognitive rehabilitation.
- Neural Implants and Restoration: Developing implantable devices that restore sensory perception and motor function by interfacing directly with neural circuits, opening doors for enhanced recovery.
- Virtual Reality and Immersive Environments: Utilizing immersive virtual reality environments to create engaging and interactive rehabilitation scenarios, enhancing both cognitive and motor interventions.
- Data-Driven Personalized Treatment: Leveraging AI and machine learning to analyze vast patient datasets, tailoring rehabilitation plans to individual needs and predicting optimal interventions.
- Home-Based Rehabilitation Solutions: Expanding home-based rehabilitation options by integrating wearable technologies, telehealth platforms, and remote monitoring for extended and convenient care.
- Interdisciplinary Collaboration: Strengthening collaboration among clinicians, engineers, data scientists, and ethicists to create holistic approaches that address neurological challenges from multiple angles.
Challenges:
- Technological Integration and Accessibility: Ensuring that cutting-edge technologies, such as AI, robotics, and BCIs, are accessible to diverse patient populations, including those with limited resources.
- Ethical Considerations: Addressing ethical challenges related to data privacy, informed consent, and potential risks associated with emerging technologies in neurorehabilitation.
- Long-Term Sustainability: Establishing sustainable models for long-term neurorehabilitation care, including funding, reimbursement, and maintenance of advanced technologies.
- Personalization Complexity: Navigating the intricate balance between standardized rehabilitation protocols and highly personalized interventions to optimize outcomes.
- Clinical Validation and Evidence-Based Practice: Conducting rigorous clinical trials to generate robust evidence for the efficacy and safety of novel neurorehabilitation approaches.
- Cultural and Societal Considerations: Addressing cultural and societal factors that may influence patient acceptance, engagement, and adherence to innovative rehabilitation technologies.
- Healthcare Integration and Education: Promoting awareness and education among healthcare professionals to foster seamless integration of advanced technologies into standard practice.
FAQs:
What is neurorehabilitation?
Neurorehabilitation is a specialized branch of healthcare that focuses on helping individuals recover and improve their physical, cognitive, and psychological functions following neurological injuries or disorders.
What are some common neurological injuries?
Common neurological injuries include strokes, traumatic brain injuries (TBIs), spinal cord injuries (SCIs), and neurodegenerative disorders like Parkinson’s disease and multiple sclerosis.
How does AI play a role in neurorehabilitation?
Artificial Intelligence (AI) is integrated into neurorehabilitation to analyze patient data, personalize treatment plans, predict recovery trajectories, and provide real-time feedback during therapy sessions.
What are robotic therapies in neurorehabilitation?
Robotic therapies involve the use of robotic devices, such as exoskeletons and robotic prosthetics, to assist, guide, or enhance movements during rehabilitation exercises, promoting functional recovery.
How do Brain-Computer Interfaces (BCIs) work in neurorehabilitation?
BCIs establish a direct link between the brain and external devices, enabling individuals to control robotic devices, communicate, or perform tasks by translating neural signals into actions.
What is the role of virtual reality in neurorehabilitation?
Virtual reality is used to create immersive and interactive environments for cognitive and motor rehabilitation, engaging patients in enjoyable exercises that promote recovery.
How do wearable sensors contribute to neurorehabilitation?
Wearable sensors capture movement data, providing real-time feedback, objective assessment, and progress tracking, which aid in customizing and optimizing rehabilitation interventions.
What are the benefits of personalized treatment plans in neurorehabilitation?
Personalized treatment plans address individual patient needs, optimizing rehabilitation outcomes, enhancing engagement, and tailoring interventions to specific impairments.
Can neurorehabilitation be conducted at home?
Yes, home-based neurorehabilitation is becoming more feasible with wearable technologies and telehealth platforms, allowing patients to continue exercises and receive remote guidance.
Are there ethical considerations in using AI and robotics in neurorehabilitation?
Yes, ethical considerations include ensuring patient consent, data privacy, minimizing risks, and addressing potential disparities in access to advanced technologies.
What is the future of neurorehabilitation?
The future of neurorehabilitation involves advancements in AI, BCIs, virtual reality, personalized treatment, interdisciplinary collaboration, and expanded home-based care to enhance recovery outcomes.
How can I find a neurorehabilitation program or facility near me?
You can consult with your healthcare provider or specialist to inquire about available neurorehabilitation programs or facilities in your area. They can guide you to appropriate resources and options.
Conclusion:
In conclusion, the intersection of innovative technologies such as artificial intelligence, robotics, brain-computer interfaces, and sensor integration has ushered in a new era of promise and potential in the field of neurorehabilitation. As we stand at the precipice of transformation, these advancements hold the key to personalized, data-driven, and holistic approaches that empower individuals recovering from neurological injuries to regain independence, functionality, and quality of life. With continued research, interdisciplinary collaboration, and ethical considerations, the future of neurorehabilitation is bright, offering renewed hope and opportunities for those on their journey to recovery.